The Brain’s Internal Compass is More Complex Than Once Thought
New details about how the brain senses orientation might lead to earlier Alzheimer’s detection.
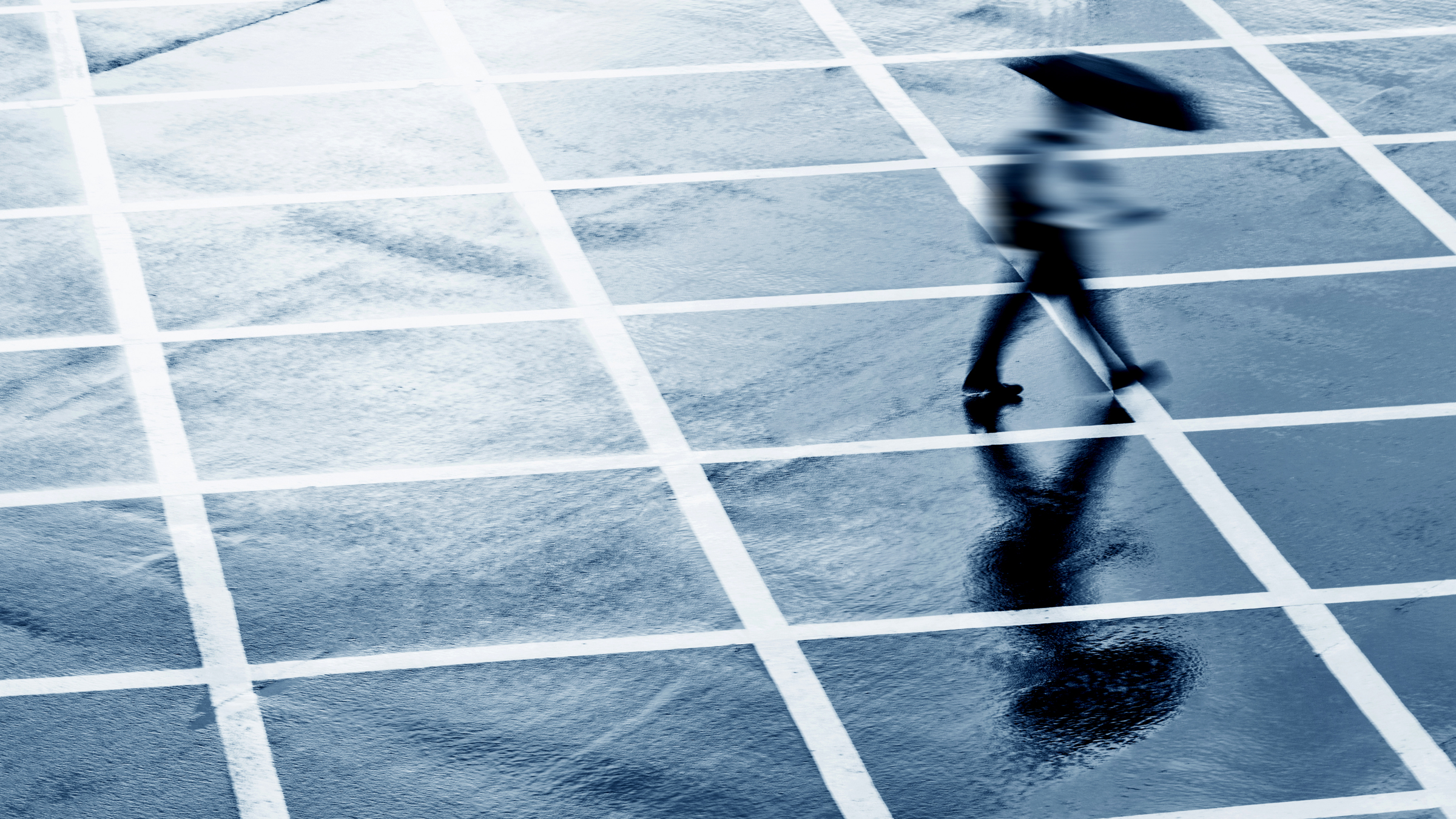
A specialized group of brain cells called head direction (HD) cells, serve as the brain’s internal compass, giving us a sense of orientation. In a new study published in the journal Nature, for the first time, neuroscientists were able to record from hundreds of HD cells simultaneously—an order of magnitude increase in comparison with prior studies. This enabled them to reveal new insights into the interactions between the internal compass and the visual environment, which has implications for disorders such as Alzheimer’s disease and for virtual reality technologies.
Study author Xue-Xin Wei, a computational neuroscientist and assistant professor at The University of Texas at Austin, developed a statistical technique for analyzing brain imaging data that enabled the team to accurately decode the internal head direction in mice with an error of only a few degrees.
“It is remarkable that, by integrating large-scale neural recording and advanced data analysis tools, we can read out the animal’s head direction from neural activity with such high precision,” said Wei.
The team, led by Mark Brandon at McGill University and Zaki Ajabi at Harvard University, discovered three things about the way HD cells function in mice.
First, when a mouse sees a confusing shift in visual information, HD cells turn down their activity, acting as a kind of “reset button” that allows them to quickly reorient themselves. Second, when a mouse sees a visual cue and then it disappears, its HD cells keep track of which direction the cue came from. The authors speculate that these memory traces may help stabilize the internal HD representation even when reliable visual cues are temporarily missing. Third, when a mouse initially sees visual cues rotating around them and then the lights go out, its HD system continues to track the apparent rotation of the visual cues. In other words, the brain has recalibrated the relationship between actual head movement and changes in internal head direction, the HD cells continue to signal in a way as if the mouse itself is rotating.
The reported findings might be relevant to the modern human experience, especially with the rapid spread of virtual reality (VR) technology. These findings “may eventually explain how VR systems can easily take control over our sense of orientation,” added Ajabi, a postdoctoral research fellow at Harvard and former Ph.D. student in Brandon’s lab.
The findings might also have significant implications for Alzheimer’s disease.
“One of the first self-reported cognitive symptoms of Alzheimer’s disease is that people become disoriented and lost, even in familiar settings,” said Brandon, an associate professor at McGill.
The researchers expect that a mechanistic understanding of how the brain’s internal compass and navigation system works might lead to earlier detection and better assessment of treatments for Alzheimer’s disease.
To test how the brain’s head direction system adapts to changing, missing or conflicting sensory information, the team placed mice in a special circular chamber with walls covered by a full 360-degree LED screen. They used a method called calcium imaging to study how brain cells in the thalamus, called HD cells, reacted to changes in visual cues on the screen in free-moving mice. At first, a vertical white line was displayed. Then, in some versions of the experiment, the line would disappear for two-minute intervals and reappear shifted 90 degrees away around the chamber, as if the world had suddenly rotated around the mouse by 90 degrees. In other versions, the line would continuously rotate around the chamber.
These latest results inspired the team to develop new computational models to better understand the underlying mechanisms. Traditionally, neuroscientists have used a type of model for describing how the HD system works called a ring attractor model. They found that their key experimental observations could be better explained by expanding such a model to incorporate “gain modulation” — changing the overall activity level in HD cells, which can act as a reset button to re-orient a confused HD system — and synaptic plasticity — the rewiring of brain circuits. In doing so, the modeling also generates new predictions that will need to be tested in future experiments.
“This work is a beautiful demonstration of how tight integrations of experimental and computational approaches can advance our understanding of neural circuit mechanisms that support behavior,” Wei said. “One interesting open question is whether the particular type of gain modulation observed in fact represents an optimal strategy for the system to control the re-orienting behavior.”
The new research was supported by the Natural Sciences and Engineering Research Council of Canada and the Canadian Institutes of Health Research.